News Story
Dimitrakopoulos, Dodson Publish Novel Modeling for Red Blood Cells in Complex Flow Systems
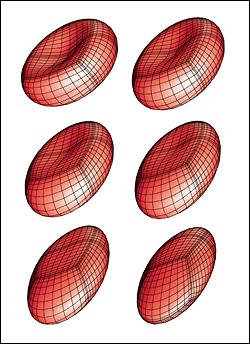
A red blood cell in a strong shear flow is gradually transformed from an initially biconcave shape into an ellipsoidal shape while its membrane tank-treads (i.e. rotates) around the cell.
Predicting how red blood cells move through the microcirculation under various conditions, and the effects their travels have on their membranes and cytoskeletons, may become easier thanks to a new model created by ChBE Associate Professor Panagiotis Dimitrakopoulos and alumnus Dr. Walter Dodson (Ph.D. '08, bioengineering, formerly advised by Dimitrakopoulos). A paper describing their work, "Tank-Treading of Erythrocytes in Strong Shear Flows via a Nonstiff Cytoskeleton-Based Continuum Computational Modeling," was published in the November 2010 issue of the top-rated Biophysical Journal. Studies on the flow dynamics of erythrocytes (red blood cells) are a challenging problem in any type of research. Experimental findings are limited by the cells' micron size and the constraints of devices that can be used to observe or measure their activity (such as specific flow-rate regimes or one view angle). In addition, the coupling of the fluid dynamics with the non-linear membrane tensions prohibits analytical solutions of the erythrocyte motion and limits the usage of the state-of-the-art three-dimensional computational methodologies. To help address the need for more effective research tools, Dodson and Dimitrakopoulos developed a computationally efficient cytoskeleton-based continuum erythrocyte algorithm. The cytoskeleton is modeled as a two-dimensional elastic solid with comparable shearing and area-dilatation resistance that follows the Skalak et. al.1 strain-hardening material law. The modeling enforces the global area-incompressibility of the spectrin skeleton (being enclosed beneath the lipid bilayer in the erythrocyte membrane) via a non-stiff, and thus efficient, adaptive prestress procedure that accounts for the locally isotropic stress imposed by the lipid bilayer on the cytoskeleton. "In the present paper we have combined our non-stiff continuum erythrocyte modeling with our spectral boundary element method for elastic capsules to solve the interfacial problem," Dimitrakopoulos explains. "However, our cytoskeleton modeling is general and can be combined with any membrane interfacial method." Dodson and Dimitrakopoulos' cytoskeleton-based modeling also provides insight on the local surface area changes of the spectrin skeleton during the tank-treading [rotation of the membrane] of the cells, complementing earlier work published of Discher et. al. in Science.2 In particular, the work shows that during tank-treading the cytoskeleton undergoes measurable local area dilatation and compression as also found experimentally via micro-pipette experiments at large static deformations by Discher et. al. References: 1 Skalak, R., A. Tozeren, R. P. Zarda, and S. Chien, Strain energy function of red blood cell membranes. Biophys. J., 13, 245-264 (1973). Return to article » 2 Discher, D. E., N. Mohandas, and E. A. Evans, Molecular maps of red cell deformation: hidden elasticity and in situ connectivity. Science, 266, 1032-1035 (1994). Return to article » For More Information: Read "Tank-Treading of Erythrocytes in Strong Shear Flows via a Nonstiff Cytoskeleton-Based Continuum Computational Modeling" (PDF) » Visit Professor Dimitrakopoulos' web site »
Published November 16, 2010