News Story
Study Reveals First Glimpse of a Light-Driven Chemical Reaction
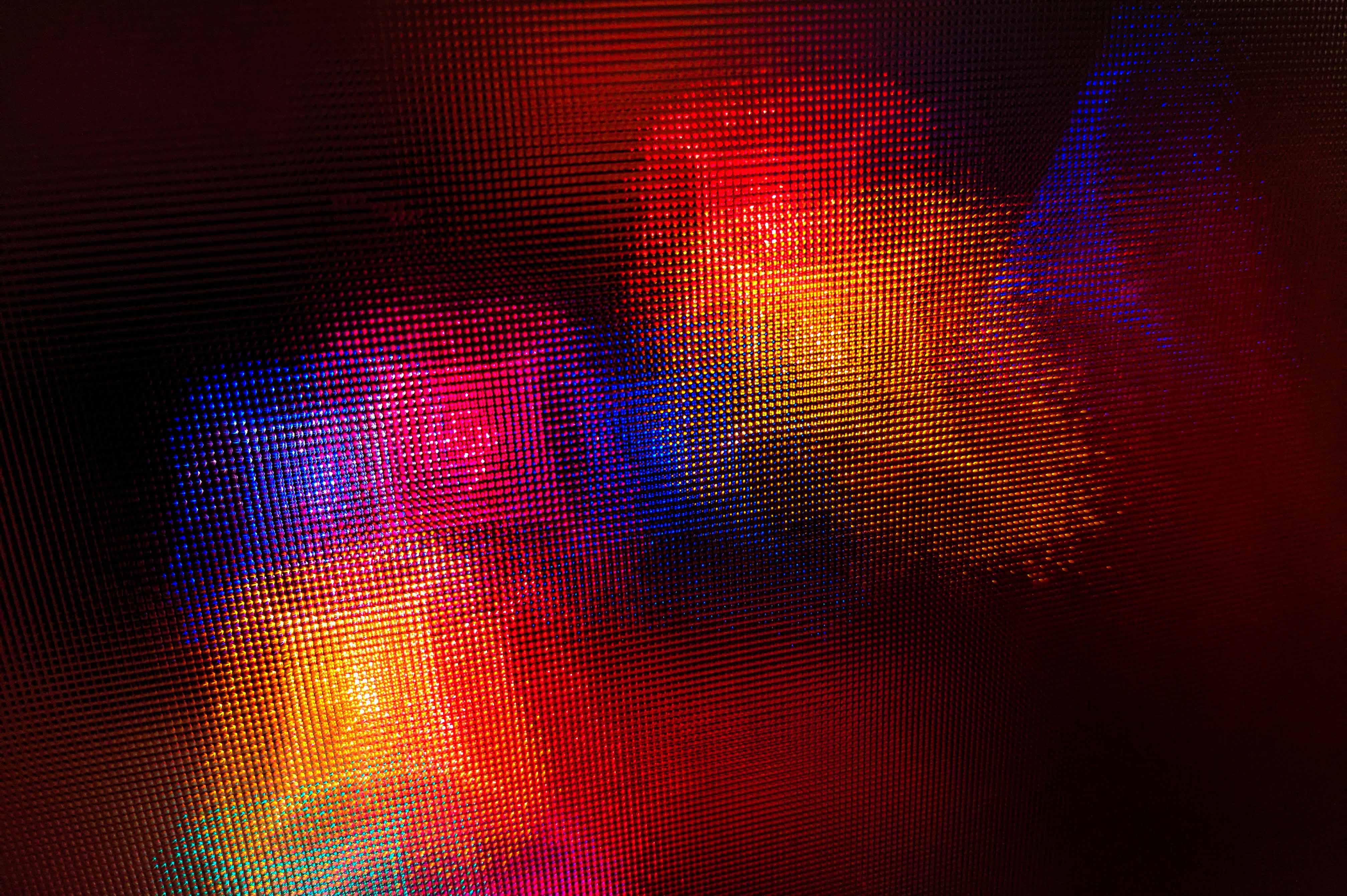
A new study revealing how light-driven reactions unfold at a microscopic level could represent a step forward in producing energy fuels with renewable processing methods.
Plasmonic photocatalysis—a process that uses light to drive chemical reactions on the surface of microscopic metals—has attracted the attention of researchers studying renewable methods to produce fuels, but previous experiments aimed at predicting how this reaction occurs have failed. On-going research co-authored by Taylor Woehl, an associate professor in the Department of Chemical and Biomolecular Engineering led to a new imaging technique involving electron microscopy, published today in the journal Matter.
Woehl and collaborators developed an advanced electron microscopy technique to image how this reaction occurs at the nanometer scale. During plasmonic photocatalysis, the interactions between particles of light (known as photons) and microscopic metals (known as plasmonic nanoparticles) are converted into chemical reactants known as “hot carriers.” Previously, it remained unclear how nanoparticle properties, like size, shape, and material, influenced how photons were converted into hot carriers.
Their study revealed how plasmonic photocatalysis occurred at the smallest scale, a fundamental breakthrough that could advance sustainable methods to generate hydrocarbon fuels. At least 24% of global greenhouse gas emissions are attributed to burning fossil fuels, according to the latest data gathered by the U.S. Environmental Protection Agency from 2019. Photocatalysis, which drives chemical reactions with light, offers an environmentally friendly alternative to burning fossil fuels, and alleviates other risks associated with their extraction and transportation.
While plasmonic photocatalysis is a potentially powerful solution to cleanly generate chemical fuels, researchers have so far lacked understanding of how this reaction unfolds at the nanometer scale. In particular, it has remained unclear how the shape and position on the nanoparticle surface influenced the transformation efficiency of light into hot carriers.
Woehl’s group demonstrated how gold nanorods convert light into hot carriers. To do this, they used a technique known as “liquid phase transmission electron microscopy,” which illuminates gold nanorods in liquid with electrons, which act as a surrogate to light, and also images the nanorods in real time. They utilized a probe reaction to directly visualize the generation of hot carriers on the nanoparticle surface, and in doing so, they became the first to observe how plasmonic photocatalysis unravels at the nanoscale.
“We were able to watch the reaction in real time, which enabled measuring how efficiently hot carriers were generated at different positions on the nanoparticle surface, which nobody had done before,” said Woehl. “Our experiments will enable establishing design rules for how to engineer more efficient photocatalysts.”
Previously, conventional methods that were used to image plasmonic photocatalysis had failed in part because they did not have enough spatial resolution to see the surface of the nanoparticles, how it changes over time, and the result of the reaction—details that prevented scientists from engineering nanoparticles for use as photocatalysts.
With time, the discovery could enable the tailored design of plasmonic nanoparticles for various applications beyond photocatalysis, including novel cancer treatments and solar absorbers.
“When light is converted into thermal energy, it will also heat up the nanoparticle’s surface. Therefore, you could embed these nanoparticles into tumor cells and illuminate them to attack the disease locally without impacting the tissue around it,” said Woehl.
This work was supported by the United States Army Combat Capabilities Development Command Army Research Laboratory (DEVCOM ARL) and the Petroleum Research Fund (PRF). Collaborators include Jonathan Boltersdorf, a research chemist at DEVCOM ARL, Amy Chen, a doctoral student in materials science and engineering and first author of the study, and Carlos A. Rios Ocampo, an assistant professor in materials science and engineering.
Published December 4, 2024